What are Quantum Computers and How Do They Work?
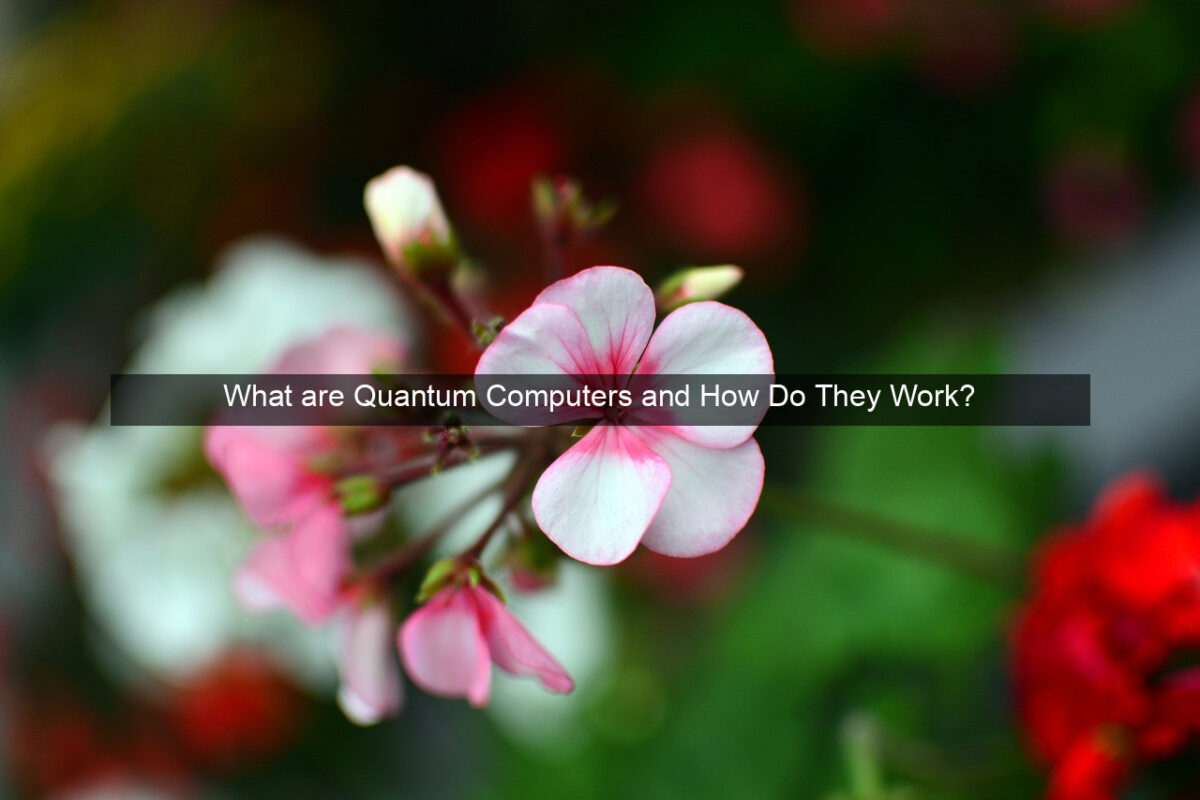
- What are Quantum Computers and How Do They Work?
- What are Quantum Computers?
- Qubits: The Building Blocks of Quantum Computation
- Superposition and Entanglement: Quantum Phenomena
- How Do Quantum Computers Work?
- Quantum Algorithms: Harnessing Quantum Power
- Quantum Gates: Manipulating Qubits
- Measurement: Obtaining Classical Results
- Challenges and Future of Quantum Computing
- Error Correction and Scalability
- Conclusion
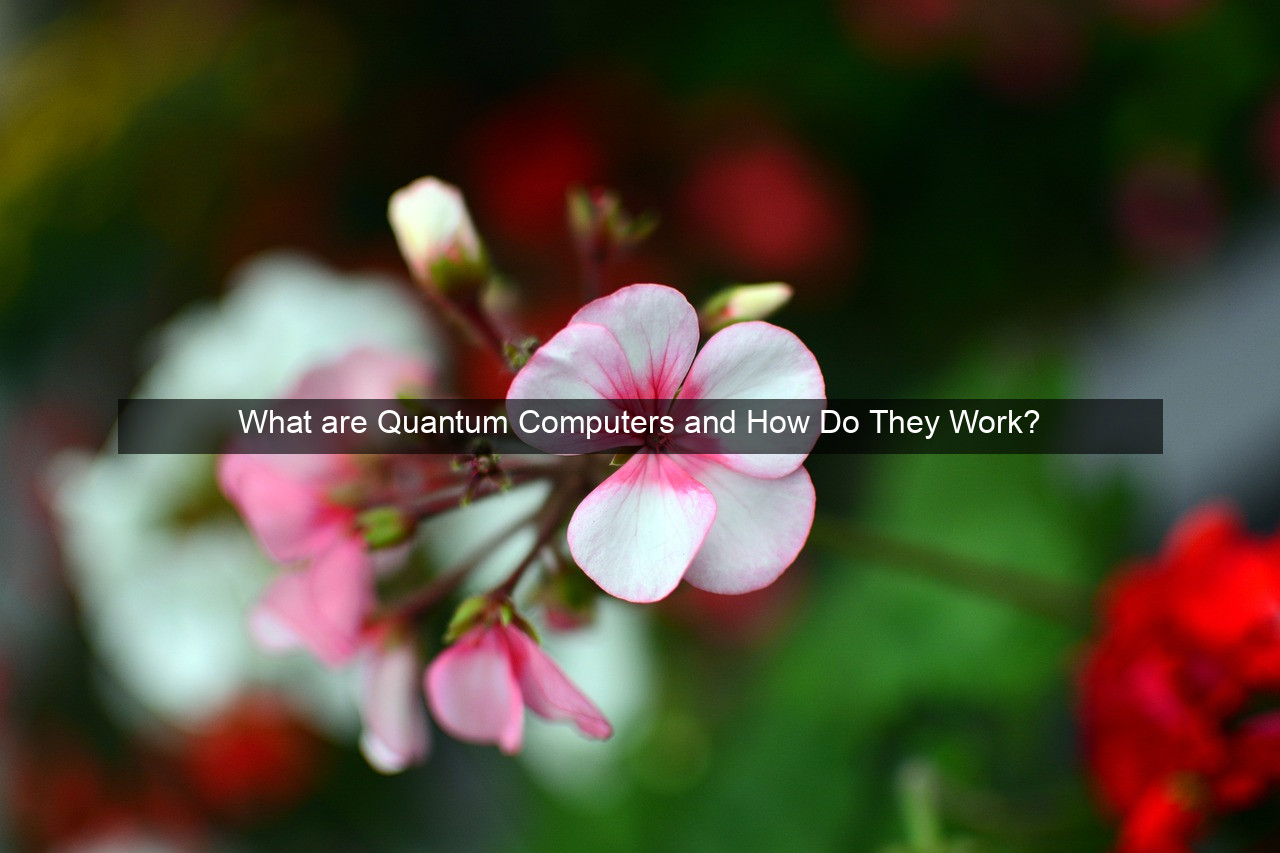
Imagine a world where complex scientific problems, like designing new drugs or modeling climate change, are solved in a fraction of the time it takes today. This is the promise of quantum computers, machines that harness the bizarre laws of quantum mechanics to perform calculations in ways classical computers can’t. These aren’t just faster versions of the computers we use now; they represent a fundamentally different approach to computation, opening doors to solving problems previously deemed intractable. But what exactly are these revolutionary machines, and how do they achieve such computational power? This article delves into the fascinating world of quantum computing, exploring its underlying principles and potential to reshape our future. From understanding qubits and superposition to exploring algorithms and the challenges ahead, we’ll unravel the mysteries behind this cutting-edge technology.
What are Quantum Computers?
Quantum computers are a new type of computer that utilizes the principles of quantum mechanics to perform powerful calculations. Unlike classical computers that rely on bits representing 0 or 1, quantum computers use qubits. Qubits can exist in a superposition, representing both 0 and 1 simultaneously. This allows quantum computers to explore multiple possibilities at once, exponentially increasing their computational power for specific tasks.

Qubits: The Building Blocks of Quantum Computation
The fundamental unit of information in a quantum computer is the qubit. Unlike classical bits, which can only be either 0 or 1, qubits can exist in a superposition of both states simultaneously. This unique property allows quantum computers to store and process exponentially more information than classical computers with the same number of bits. Think of a coin spinning in the air – it’s neither heads nor tails until it lands. A qubit is similar, existing in a probabilistic state of both 0 and 1 until measured.
Qubits can be created using various physical systems, including trapped ions, superconducting circuits, and photons. Each system has its own advantages and challenges, and researchers are actively working to improve the stability and control of qubits to build more powerful and reliable quantum computers. The stability of a qubit is crucial for maintaining its quantum state and preventing errors in computation.
Manipulating qubits involves using quantum gates, analogous to logic gates in classical computers. These gates perform operations on qubits, changing their superposition and entanglement, which is another key concept in quantum computing. Entanglement allows two or more qubits to become linked, so that even when separated, they share the same fate. Measuring one entangled qubit instantly reveals the state of the other, regardless of the distance between them.
Superposition and Entanglement: Quantum Phenomena
Superposition is the ability of a quantum system, like a qubit, to exist in multiple states simultaneously. This is a cornerstone of quantum computing, enabling qubits to perform calculations on multiple possibilities at once. Imagine a coin spinning in the air – it’s both heads and tails until it lands. A qubit in superposition is similar, existing in a probabilistic state of both 0 and 1.
Entanglement, another crucial quantum phenomenon, links two or more qubits together. Even when separated by vast distances, entangled qubits share the same fate. Measuring the state of one instantly reveals the state of the other, a phenomenon Einstein called “spooky action at a distance.” This interconnectedness is a powerful resource for quantum computation.
These two phenomena, superposition and entanglement, are what give quantum computers their immense potential. They allow for parallel processing on an unprecedented scale, enabling the exploration of a vast number of possibilities simultaneously. This is why quantum computers are expected to revolutionize fields like medicine, materials science, and cryptography.
How Do Quantum Computers Work?
Quantum computers leverage the principles of superposition and entanglement to perform computations in a fundamentally different way than classical computers. Instead of processing bits sequentially, they manipulate qubits, which can exist in multiple states at once. This allows them to explore a vast number of possibilities simultaneously, leading to exponential speedups for certain types of problems.
Quantum Algorithms: Harnessing Quantum Power
Quantum algorithms are specifically designed to exploit the unique capabilities of quantum computers. These algorithms utilize superposition and entanglement to solve problems that are intractable for classical computers. Shor’s algorithm, for instance, can factor large numbers exponentially faster than any known classical algorithm, posing a threat to current encryption methods.
Grover’s algorithm, another important quantum algorithm, provides a quadratic speedup for searching unsorted databases. While not as dramatic as Shor’s algorithm, it still offers significant advantages for large datasets. Developing new quantum algorithms is a crucial area of research, as it unlocks the full potential of quantum computing.
Quantum algorithms are still in their early stages of development, and researchers are actively exploring new ways to harness the power of quantum mechanics for computation. As quantum computers become more powerful and stable, we can expect to see even more innovative and impactful algorithms emerge.
Quantum Gates: Manipulating Qubits
Quantum gates are the building blocks of quantum circuits, analogous to logic gates in classical computing. These gates perform operations on qubits, changing their state and entanglement. They are essential for manipulating qubits and performing complex computations. Just like logic gates manipulate bits, quantum gates manipulate qubits, enabling the creation of complex quantum algorithms.
Different types of quantum gates exist, each performing a specific operation. Hadamard gates, for example, create superposition, while CNOT gates create entanglement. The precise sequence of quantum gates applied to a set of qubits determines the computation performed. Understanding and controlling these gates is crucial for building and operating quantum computers.
The development of new and more efficient quantum gates is an active area of research. As quantum computers scale up, the ability to precisely control and manipulate qubits becomes increasingly important for achieving accurate and reliable results.
Measurement: Obtaining Classical Results
While quantum computers operate on qubits in superposition, the final output must be a classical result, a sequence of 0s and 1s. This is achieved through measurement, which forces the qubit to collapse from its superposition into a definite state, either 0 or 1. The act of measurement fundamentally alters the quantum state, forcing it to choose a definite value.
The probabilistic nature of quantum mechanics means that the outcome of a measurement is not always deterministic. Repeated measurements on the same qubit may yield different results, governed by the probabilities encoded in the qubit’s superposition. Understanding these probabilities and how they relate to the computation being performed is essential for interpreting the results of a quantum computation.
The measurement process is a crucial step in quantum computing, bridging the gap between the quantum world and the classical world where we interpret and utilize the results. Improving measurement techniques is vital for increasing the accuracy and reliability of quantum computations.
Challenges and Future of Quantum Computing
Quantum computing is a rapidly evolving field, but it still faces significant challenges. Building and maintaining stable qubits is extremely difficult, requiring highly specialized equipment and environments. The coherence time of qubits, the duration they can maintain their quantum state, is limited, making long computations challenging. Overcoming these technical hurdles is crucial for the advancement of quantum computing.
Error Correction and Scalability
Quantum computers are highly susceptible to errors due to noise and decoherence. Developing robust error correction techniques is essential for building reliable and scalable quantum computers. As the number of qubits increases, so does the complexity of managing and correcting errors. Scalability, the ability to build larger and more powerful quantum computers, is another major challenge.
Current quantum computers are limited in the number of qubits they can effectively control. Scaling up the number of qubits while maintaining their stability and coherence is a significant technological hurdle. Overcoming these challenges is crucial for realizing the full potential of quantum computing.
Researchers are exploring various approaches to error correction and scalability, including topological qubits and new quantum architectures. These advancements are paving the way for larger and more fault-tolerant quantum computers in the future.
Conclusion
Quantum computing is a revolutionary technology with the potential to transform various fields. While still in its early stages, the progress made in recent years is remarkable. Overcoming the challenges of scalability and error correction will pave the way for more powerful and reliable quantum computers, unlocking their full potential to solve some of the world’s most complex problems.
What is a quantum computer?
A quantum computer is a type of computer that uses quantum mechanics to perform computations. They utilize qubits, which can represent both 0 and 1 simultaneously, allowing them to explore multiple possibilities at once.
What is a qubit?
A qubit is the basic unit of information in quantum computing. Unlike a classical bit,